Search
Mechanical and Fluid Systems

Passive Fuel Cell Surface Power System (PaCeSS)
NASA’s envisioned Lunar and Martian surface operations will require constant and reliable power systems. Traditional power architectures, including solar cells and batteries, cannot be solely relied upon due to the lengthy lunar nights and challenging thermal environments. How-ever, fuel cells, including primary fuel cells and regenerative fuel cells, represent a promising means for energy generation and storage on planetary and lunar surfaces.
PaCeSS could further improve mission flexibility by significantly enhanc-ing reliability and longevity with fully passive fuel cell power generation capability. Test systems have been built to validate the performance characteristics of various PaCeSS technology elements, and many of the component materials have already been characterized. Some of these novel technology elements already demonstrated include a two-phase thermosyphon operation in fuel cell conditions, a passive shape memory actuator operation using two-phase water, and a shape memory alloy radiator turndown.
Although the current design of the shape memory alloy actuated rad-iator system is dependent on partial gravity and space-like environments where heat rejection is performed primarily via radiation, there may be ways of using the same basic system for controlling fuel cell temper-ature via convective heat rejection for terrestrial applications. Addition-ally, other elements of this concept could be commercialized terrestri-ally, including the thermosyphon heat transport mechanism, a multi-purpose vapor chamber, and a thermal management system that uses water by-product as the thermal management medium.
The Passive Fuel Cell Surface Power System is at a technology readiness level (TRL) 3 (analytical and experimental critical function and/or characteristic proof of concept) and is now available for patent licensing. Please note that NASA does not manufacture products itself for commercial sale.
Power Generation and Storage
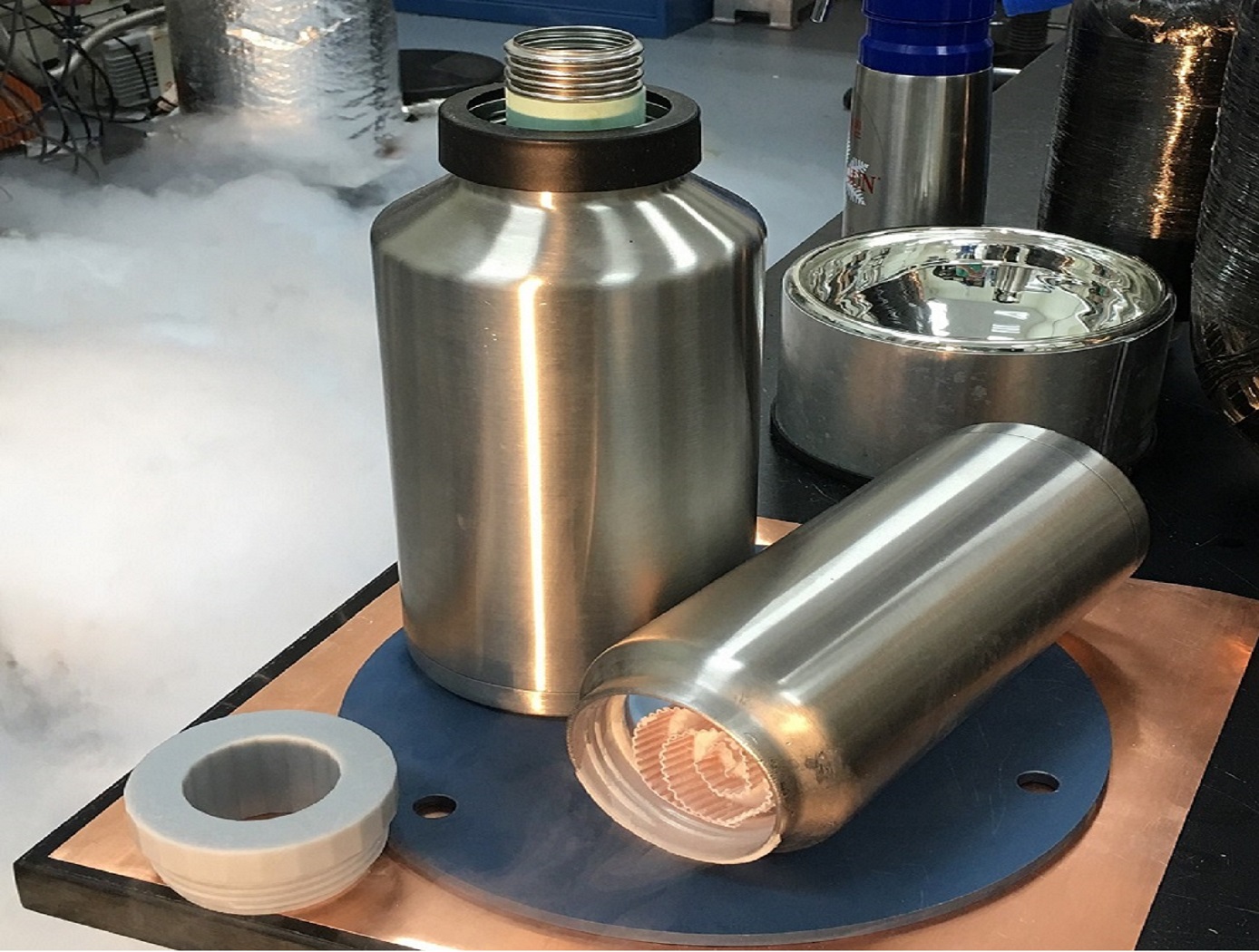
Cryogenic Flux Capacitor
Storage and transfer of fluid commodities such as oxygen, hydrogen, natural gas, nitrogen, argon, etc. is an absolute necessity in virtually every industry on Earth. These fluids are typically contained in one of two ways; as low pressure, cryogenic liquids, or as a high pressure gases. Energy storage is not useful unless the energy can be practically obtained ("un-stored") as needed. Here the goal is to store as many fluid molecules as possible in the smallest, lightest weight volume possible; and to supply ("un-store") those molecules on demand as needed in the end-use application. The CFC concept addresses this dual storage/usage problem with an elegant charging/discharging design approach.
The CFC's packaging is ingeniously designed, tightly packing aerogel composite materials within a container allows for a greater amount of storage media to be packed densely and strategically. An integrated conductive membrane also acts as a highly effective heat exchanger that easily distributes heat through the entire container to discharge the CFC quickly, it can also be interfaced to a cooling source for convenient system charging; this feature also allows the fluid to easily saturate the container for fast charging. Additionally, the unit can be charged either with cryogenic liquid or from an ambient temperature gas supply, depending on the desired manner of refrigeration. Finally, the heater integration system offers two promising methods, both of which have been fabricated and tested, to evenly distribute heat throughout the entire core, both axially and radially.
NASA engineers also applied the CFC to a Cryogenic Oxygen Storage Module to store oxygen in solid-state form and deliver it as a gas to an end-use environmental control and/or life support system. The Module can scrub out nuisance or containment gases such as carbon dioxide and/or water vapor in conjunction with supplying oxygen, forming a synergistic system when used in a closed-loop application. The combination of these capabilities to work simultaneously may allow for reduced system volume, mass, complexity, and cost of a breathing device.
Power Generation and Storage

Next Generation “Closed Strayton” Engine Design
The core “Strayton” generator technology consists of a gas turbine engine with short, axial pistons installed inside the hollow turbine shaft. These pistons form a Stirling engine that cycles via thermo-acoustic waves, transferring heat from the turbine blades to the compressor stage, which improves overall engine performance. Power to an alternator is, thus, delivered from both turbine shaft rotation and the oscillation of the internal pistons.
This synergistic relationship is markedly enhanced in a closed-cycle system, where the sealed turbine engine recirculates a working fluid heated via an external source, such as a hydrogen fuel cell and combustor. This system supports higher compression ratios, reduces the turbine diameter to less than 4”, and eliminates the need for large recuperators. Operational efficiency is projected to extend into the low temperature range (750° C), reducing the need for advanced materials and providing cleaner combustion for hydrogen-based applications. Pressurized, inert working fluids also replace mechanical bearings and gearboxes, enabling years of maintenance-free operation.
The fuel cell and Stirling cycle produce 10% of the total system energy, while the Brayton cycle produces 90%. Other external heat sources could include nuclear, solar, or biogas. Conservative estimates for the hydrogen fuel-cell configuration lifetime are in the 100,000 hour range.